Ceramic matrix composites (CMC), based on reinforcements of carbon fibers and matrices of silicon carbide (called C/SiC or C/C-SiC composites) represent a relatively new class of structural materials. In the last few years new manufacturing processes and materials have been developed. Short fiber reinforcements, cheap polymer precursors and liquid phase processes reduced the costs by almost one order of
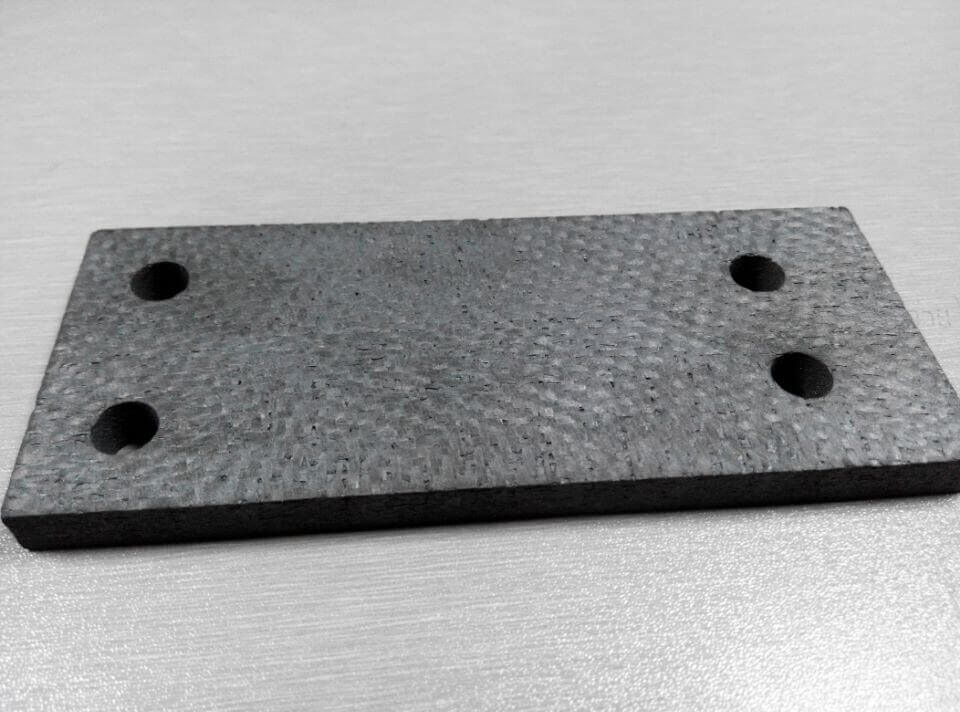
C-SIC
magnitude in comparison to first generation C/SiC composites which were originally developed for space and military applications. Besides high mass specific properties and high thermal stability, functional properties like low thermal expansion and good tribological behaviour play an increasing importance for new commercial applications like brake disks and pads, cluthches, calibration plates or furnace charging devices.
First non-oxide CMCs, based on carbon/carbon composites, were developed in the 1970s as lightweight structures for aerospace applications. They had to be designed as limited life structures as the environmental conditions were highly aggressive and the longterm behavior of these composites was still unknown. Typical representatves for such components were rocket nozzles, engine flaps, leading edges of spacecraft and brake disks of aircraft. Their lifetime comprises several minutes to some few hours under highest thermomechanical requirements which can not be fulfilled by any other structural material.
In order to improve the oxidation resistance and thus the application lifetime of these composites, reserach has been exerted on using ceramics instead of carbon as the matrix material. Silicon carbide is particularly suitable as a matrix material due to its high oxidation
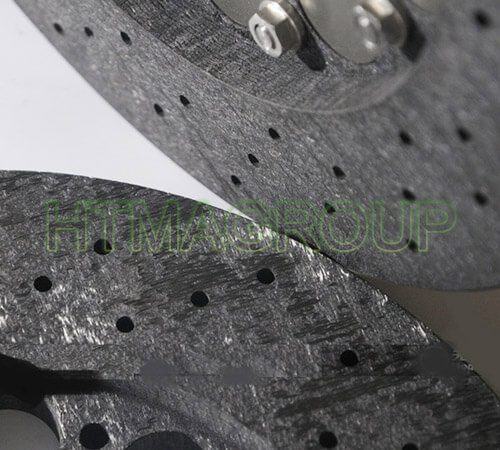
carbon ceramic composite
resistnce, its superior temperature and thermal shock stability and its high creep resistnce. Practically, similar manufacturing techniques can be used for the silicon carbide matrix formaton of C/SiC composites as for the manufacture of carbon/carbon composites. Generally, ceramic matix composites have been developed to combine the advantageous properties of monolitic ceramics with a high damage tolerance, which is known for example from the reinforcing of fiber reinforced polymers. However, the mechanisms which cause high damag tolerance are completely different for both classes of material. Polymers are reinforced with strong and stiff fibers, whereas the matrix is weak and of low strength, stiffness as well as thermal stability. A strong bonding between matrix and fibers is desired as a result of high fiber surface reactions. Based on the differences of stiffness between fibers and polymer, the matrix itself is stressed only slightly and the energy release rate of a matrix crack is low because of the modest matrix strength. Therefore, the highly loaded fibers are able to stop cracks without being damaged.
Ceramic matrix composites are characterized by the fact that the stiffness of both, fibers and matrix, are in the same order of magnitude. High fiber/matrix bonding forces result in stresses which are similar for the matix as well as for the fibers and the damage tolerance is comparable low to monolithic ceramics. The opposite case with extremely low fiber/matrix bondings leads to a nearly stress-free matrix and a high fracture toughness. However, as the debonding and shear properties mainly depend on the frictional effects, such kind of composites are usually not suitable as a structural material. Damage tolerant CMCs therefore require moderate fiber/matrix bondings with adapted interphases. The interphase microstrucre can vary from sharp, non-reactive interfaces to in situ reacted interfaces, porous or multilayer interfaces and is responsible for the stopping and deflecting of matrx cracks.
Similar to polymer and metal matrx composite, the CMC’s fracture hebaviour and properties are dominated by the reinforcting fibers. But to an even higher degree the fibers must show a high stiffness and an extreme thermal stability. Carbon fibers fulfill these requirements in an outstanding way. They are commercially available in various modifications, weavable to preforms and carbon fibers show a very high thermal stability well above 2000°C. However, their main disadvantage is the degradation in an oxidising atmosphere beyond 450°C, resulting in the need for an external oxidation protection. It is known from from oxidation kinetics that increasing the final heat treatment temperature results in an improved oxidation resistance of the C-fibers. Therefore, the oxidation resistance is also improved by reinforcements with high modulus or altra high modulus carbon fibers in comparison to high tenacity fibers.
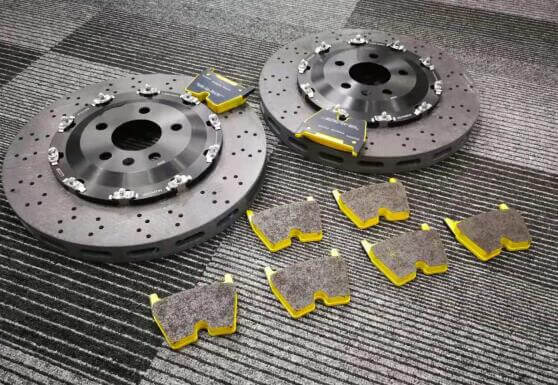
carbon ceramic composite (C-SiC) brakes for racing car-2
Long-term oxidation protection requires multilayers protection coatings, where the carbon/carbon or carbon/silicon carbide composite is protected for example with SiC layers and additional self-heating glass forming layers, based on oxides like mullite, alumina or silicon. The silicon carbide layer can be performed via pack cementation, but superior oxidaton resistance can be achieved with pure β-SiC layers, deposited via the CVD process.
Due to the anisotropic coefficient of thermal expansion of C/C, C/SiC and C/C-SiC, the oxidation protection of these composites is more difficult than it is for non-reinfroced carbon or grapite bulk materials. The mismatch of CTE between the CVD-SiC coating and the carbon fiber reinforcement creates cracks in the SiC coating during the cooling-down period after deposition. Crack formation starts at approximately 100°C below the CVD coating temperature. Therefore, CVD-SIC coated composites show the highest oxidaton rate about 800°C, the maximum between crack opening and oxidation kinetics. As a result, sophisticated oxidaton and corrosion coatings can only reduce the material degradation in a certain temperature interval under static conditions, but all available protection coatings are not able to prevent oxidation completely under dynamic conditions. The designers ntd users of C/SiC and C/C-SiC composites have to keep these inherent restrictions under consideration in order to apply these materials adequately, resulting for example in short inspection periods and higher efforts in in-service monitoring by NDE methods.
Related news /knowledge:
- Carbon fiber reinforced silicon carbide composites (C/SiC, C/C-SiC)-(2)-application
- Carbon fiber reinforced silicon carbide composites (C/SiC, C/C-SiC)-(3)-application
- Carbon fiber reinforced silicon carbide composites (C/SiC, C/C-SiC)-(6)-processing
- Carbon fiber reinforced silicon carbide composites (C/SiC, C/C-SiC)-(7)-processing